The biomechanics of gymnastics can quickly become confusing despite gymnasts making the skills look so easy. Gymnastics coaches should have a good understanding of biomechanics and be able to analyze even the most basic of gymnastics moves in order to develop athletes to their full potential.
In this article, we will delve into the science of gymnastics and explore the physics and biomechanics behind our sport.
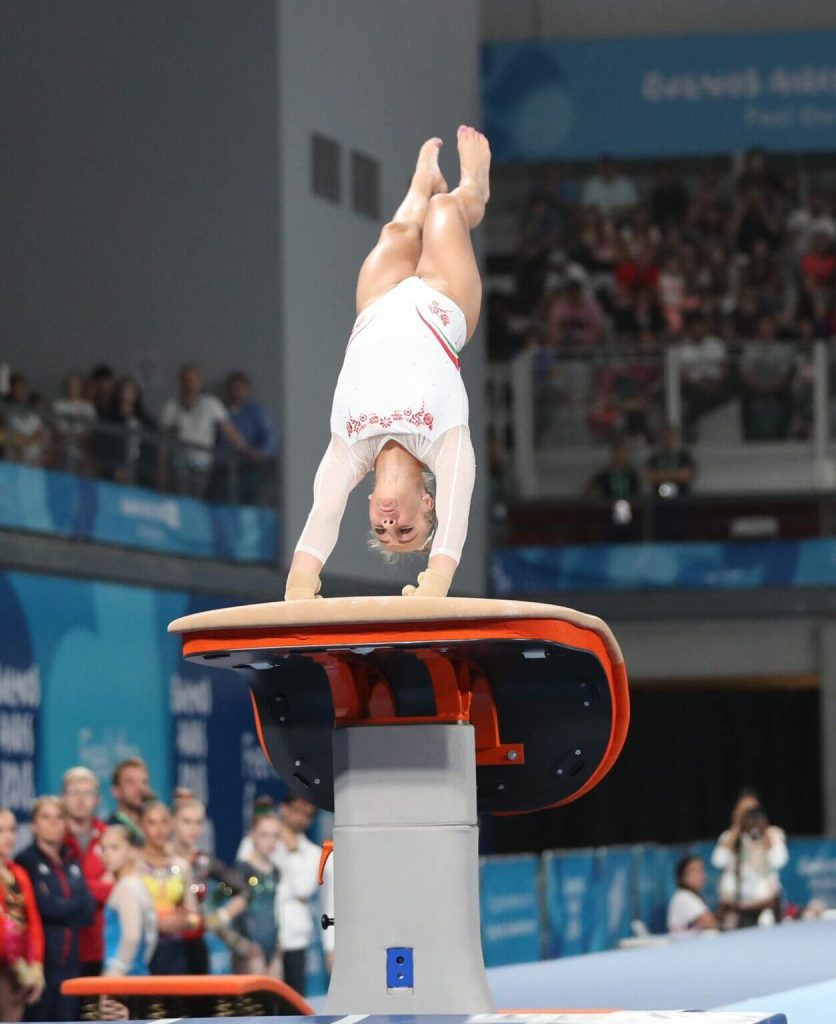
Physics and Gymnastics
It is easy to be put off by complicated scientific jargon when it comes to physics, however, the aim of this article is to provide a straightforward explanation that can be understood by anyone with an interest in gymnastics.
Where I do use scientific terms there will also be examples of how they can be applied in gymnastics in ‘real life’ examples.
Forces
The term ‘Forces’ in physics means anything that causes an object to have a change in motion. This could be a start or stop, a change in direction or a change in the rate of movement.
Gravity is a force that causes a gymnast to fall or drop to the ground after they jump in the air. Although gymnasts are referred to as ‘defying gravity’ unfortunately this is not actually possible!
Friction is another force that affects gymnasts. Friction is created when two surfaces contact each other. For example, a gymnast’s hands make contact with the vault. If the hands are sweaty it will reduce the amount of friction and cause the gymnast to slip off. This is why some gymnasts chalk their hands.
Muscular Force is controlled by the person themselves so it’s referred to as an internal force. A gymnast can decide how much force they put into something and when.
Air Resistance is an external force that has minimal impact on gymnasts because the sport is mostly practiced indoors where there is very little wind or anything that will disrupt a gymnast performing. It’s also one of the reasons why gymnasts wear tight-fitting leotards rather than baggy clothes which could increase air resistance when moving.
Forces are measured in Newtons (n). One newton is equivalent to the force needed to move one kilogram an acceleration of one meter per second. As you probably realize already Newtons are named after Sir Isaac Newton, but more on him and Newton’s Three Laws later in the article.
Momentum
Momentum is a measure of an object’s motion, and it is defined as the product of its mass and velocity. An object’s momentum is important because it tells us how much force is required to change its motion. Specifically, if a force is applied to an object, it will cause a change in its momentum, which is known as an impulse.
When a gymnast performs a run-up for a vault or a tumbling pass, they build up momentum by accelerating themselves to a high speed. This momentum is then used to launch themselves off the springboard or perform a series of flips and twists in the air. Crucially when a gymnast is at rest, for example standing still at the start of the run-up, they have no momentum.
Center of Gravity
The center of gravity refers to the imaginary point where the force of gravity appears to act on an object. It is the point at which an object’s weight is evenly distributed in all directions. The center of gravity is calculated by taking the weighted average of the positions of all the individual parts of the object.
When a gymnast is standing with their hands by their side, the center of gravity will be around the navel. But when the gymnast lifts their arms above their head the center of gravity moves upwards towards the rib cage.
Stability
Understanding where the center of gravity lies is essential for stability. For example on landings gymnasts often spread their feet, raise their arms and bend their knees when landing, as this helps keep their center of gravity over their base of support.
The base of support in this case would be the roughly rectangle-shaped area between the gymnast’s two feet. If an imaginary line ran straight down from the gymnast’s center of gravity, it would hit the floor in the middle of that base, therefore giving the gymnast stability.
If the gymnast dropped their chest down too far on the landing, it would move the center of gravity forwards, and if it moved outside the base of support, the gymnast would become unstable and either fall or need to adjust their foot position.
Biomechanics in Gymnastics
Biomechanics is the study of motion based on mechanics, principles of physics and human anatomy. When we think about sports such as gymnastics biomechanics becomes really useful for many reasons. These are the main areas of biomechanics, that when understood and applied to a gymnasts performance, will improve their technique.
Angular Motion
Angular motion refers to the motion of an object around a fixed point or axis, where the object moves in a circular path. This type of motion is also known as rotational motion.
Angular motion plays a crucial role in gymnastics, as many of the skills and movements performed by gymnasts involve rotation around an axis or fixed point. For example, when a gymnast performs a somersault or roll, they rotate their body around an imaginary point (axis) somewhere near their core. Similarly, when they perform a spin on the beam or floor, they should rotate around a vertical axis that runs from head to toe.
Newtons First Law
Newton’s first law, also known as the law of inertia, states: “An object at rest stays at rest and an object in motion stays in motion with the same speed and in the same direction unless acted upon by an unbalanced force.”
In the context of gymnastics, this law can be seen in action when a gymnast is performing a stationary hold such as a handstand. The gymnast’s body is at rest and will remain at rest unless acted upon by an external force, such as gravity or muscle fatigue. Once the gymnast decides to come out of the handstand, they must apply an unbalanced force to initiate movement. Similarly, when a gymnast is performing a tumbling pass, they will continue moving forward with the same speed and direction unless acted upon by an external force such as air resistance, friction or a landing mat. The application of an unbalanced force such as a handstand block or a landing technique is needed to change the motion of the gymnast’s body.
Newtons Second Law
Newton’s second law states: “The acceleration of an object is directly proportional to the force applied to it and inversely proportional to its mass.”
In the context of gymnastics, this law can be observed when a gymnast is performing a skill that requires a large amount of force, such as a vault. According to the second law, the amount of acceleration produced by the gymnast’s body is directly related to the force they apply to the springboard or vaulting table, and inversely related to their body mass. This means that the more force the gymnast applies to the apparatus, the greater their acceleration will be. Additionally, a lighter gymnast will experience a greater acceleration for the same amount of force applied compared to a heavier gymnast.
The second law can also be applied to skills such as a back handspring, where the gymnast uses their muscles to generate force to propel their body into the air. In this case, the amount of acceleration the gymnast experiences is directly related to the amount of force they generate with their muscles and inversely related to their body mass. The stronger the gymnast’s muscles, the greater the force they can produce, resulting in higher acceleration and a more powerful back handspring.
Overall, Newton’s second law helps us understand the relationship between force, acceleration, and mass, and how these factors influence the performance of gymnastics skills.
Newtons Third Law
Newton’s third law states: “For every action, there is an equal and opposite reaction.”
In the context of gymnastics, this law can be seen when a gymnast is performing any type of movement that involves interacting with their surroundings, such as a cartwheel or a release skill on bars. According to the third law, when the gymnast applies a force to the ground or a piece of equipment, the ground or equipment will apply an equal and opposite force back to the gymnast’s body. This means that if a gymnast pushes down with their feet to perform a cartwheel, the ground will push back up with an equal force, propelling the gymnast forward and allowing them to complete the skill.
Similarly, in a release skill on bars, when the gymnast pushes away from the bar to release themselves, the bar will push back with an equal and opposite force, propelling the gymnast away from the bar and allowing them to perform the release. The third law applies to all movements in gymnastics and helps us understand how the interaction between the gymnast and their surroundings influences their performance.
Moment of Inertia
The moment of inertia describes how difficult it is to rotate an object around a particular axis.
Moment of inertia is a measure of an object’s resistance to rotational motion. It is determined by the object’s mass distribution and the distance between the object’s axis of rotation and the mass. In simpler terms, the moment of inertia describes how difficult it is to rotate an object around a particular axis.
In gymnastics, the moment of inertia is a crucial concept as it can determine the success of certain moves. For example, consider a gymnast performing a giant swing on uneven bars. As the gymnast rotates around the bar, their moment of inertia plays a key role in determining the speed and stability of the swing. If the gymnast’s body is tightly tucked in, their moment of inertia is reduced, making it easier for them to rotate faster. However, if their body is more spread out, their moment of inertia is increased, making it more difficult to maintain the swing.
However, in the case of swings on the uneven bar, gymnasts are expected to perform it in a straight position, so the rules may affect the final skill as well!
Glossary
Here are some other bio mechanical terms which are often used in gymnastics and their definitions.
Acceleration
acceleration refers to the changes in the speed and/or direction of movement of a body part or the whole body. When a body undergoes acceleration, its velocity (speed and direction) changes. The acceleration of a body can be positive, negative, or zero.
Conservation of Energy
Conservation of energy is the principle that energy cannot be created or destroyed, only transferred or transformed from one form to another. In the context of gymnastics and biomechanics, conservation of energy means that the total amount of energy in a system remains constant, despite any internal changes that may occur within the system.
For example, when a gymnast performs a floor routine, they convert their potential energy (energy stored in their body due to their position above the ground) into kinetic energy (energy of motion) as they execute their movements. However, the total amount of energy in the system remains constant throughout the routine, and none of the energy is lost or created during the performance, only transferred into the floor or mats beneath the gymnast when they land.
Inertia
Inertia is the tendency of an object to resist a change in its state of motion. In simpler terms, it means that an object will continue to stay at rest or keep moving in a straight line with a constant speed unless a force is applied to it. The greater an object’s mass, the greater its inertia, which means it will be harder to change its motion.
Joint Mechanics
The joints in the body play a crucial role in gymnastics. The range of motion and stability of the joints affect the ability of a gymnast to perform certain moves.
Torque
Torque is a twisting force that causes rotation. In gymnastics, it is one possible way to create and control angular motion during flips and twists.
Kinetics
Kinetics is the study of forces that cause motion. In gymnastics, kinetics is used to analyze the forces that affect gymnasts during their movements.
Biomechanical Analysis of Gymnastics Moves
Biomechanical analysis can be used to understand the mechanics behind specific gymnastics moves. This is extremely useful when a gymnast struggles to perform a certain move.
For example, if a gymnast performs a front somersault but lands on their back rather than feet, most people would rightly notice that the gymnast under rotated and only completed 3/4’s of the somersault.
Using biomechanical principles we then need to figure out how to make the gymnast try and rotate more. Possible fixes would be:
- Tuck more in the somersault. The smaller the shape, the greater the rotation.
- Tuck faster. The quicker the pulling action into the tuck shape, the greater the rotation.
- Jump higher in the take-off. The greater the height, the more time to allow the rotation to happen.
Gymnastics is a tough sport so along with these possible solutions, the gymnast may need to build up strength or flexibility in order to be able to jump higher or tuck more.
That’s why it’s important to be taught gymnastics in a professional facility with a coach that understands biomechanics to a good level. Self-taught gymnasts will find it difficult (and potentially dangerous) to attempt advanced skills and recognize what they need to improve on.
Conclusion
The biomechanics of gymnastics involves a great deal of physics and science so study it in detail to improve your understanding, especially if you are a coach or thinking of becoming a gymnastics coach. By understanding the science behind gymnastics, both gymnasts and coaches alike can work to improve performance and prevent injuries.
Interested in learning more about the biomechanics of gymnastics? Book for a free trial session at Synergy here.
Sources
- A Complete Guide to Gymnastics Hand RipsAre you tired of dealing with painful gymnastics rips on your hands from training? Look no further – this article offers a comprehensive approach to… Read more: A Complete Guide to Gymnastics Hand Rips
- Is Gymnastics Dangerous? (Facts and Comparisons)Gymnastics is acknowledged as a highly technical and physically demanding sport. It inherently carries a risk of injury, which is why most coaches and clubs… Read more: Is Gymnastics Dangerous? (Facts and Comparisons)
- The Fascinating Evolution of Gymnastics (History and Facts)Gymnastics is a sport that has been around for centuries and has evolved significantly throughout history. From its origins in ancient Greece to the modern-day… Read more: The Fascinating Evolution of Gymnastics (History and Facts)
- The Ultimate Parallel Bars Skills ListParallel bars are one of the most challenging events in men’s gymnastics. Mastering the right moves first on parallel bars is essential to improving your… Read more: The Ultimate Parallel Bars Skills List
- The Long-Term Effects of Gymnastics on the Body (A Complete Guide)While gymnastics can offer many benefits, such as improved fitness and self-confidence, many people also ask what are the Long-Term Effects of Gymnastics on the… Read more: The Long-Term Effects of Gymnastics on the Body (A Complete Guide)
- What Age Does Competitive Gymnastics Start?Ever wondered what age does competitive gymnastics start? It’s well known that gymnasts start training at a young age to develop the skills and strength… Read more: What Age Does Competitive Gymnastics Start?